Biomineralised
Bacterial
Cellulose
Binanox
iGEM Leiden
2022
Creative Fields
Nanobiology
Biomaterials
Sustainability
Responsibilities
Experimental Design
Data Analysis
Science Communication
Location
Delft, NL
Year
2022
Improving water resistance of biomineralised bacterial cellulose with bio-based
Keywords
Biomaterials, sustainability, self-assembly, cellulose, nacre
Bacterial cellulose’s purity and structure allows it to tangle around calcium carbonate crystals to form a tough material. This is achieved by a three step formulation process facilitated by three different bacteria. However, one of the pressing issues preventing this material from widespread adoption is its capacity to absorb water. Bacterial cellulose absorbs up to 150x of water mass, which leads to the disruption of the material’s excellent mechanical properties. My objective was to find adequate techniques to improve the material’s water resistance.
Biomineralised Bacterial Cellulose
It is challenging to find good combinations of mechanical and physical properties
within bio-based materials. Mechanical properties, such as high tensile strength, toughness, and strain, and physical properties, such as thermostability, hydrophobicity, and permeability, rarely coexist in natural materials. Considering the lack of natural materials with a good combination of properties similar to plastics, scientists have given attention to producing novel composites with readily and plentifully available biological matter. For example, Yu et al., 2021 discusses an easily scalable self-assembly approach for producing biomineralized bacterial cellulose (BMBC) composite in the recently published paper. This material is synthesised through three fermentations, and it consists of microfibrillated bacterial cellulose (BC), poly-gamma-glutamic acid (PGA), and crystals of CaCO3 and MgCO3. The obtained material’s mechanical properties resemble nacre and acrylonitrile butadiene styrene (ABS) plastic (see Table 1 in Yu et al., 2021 for a comparison). However, this material demonstrates some drawbacks in its physical properties.
One of the deficient properties is the high hydrophilicity of this material, which can be attributed to the high content (40%) of the very hydrophilic bacterial cellulose in the composite. When BMBC absorbs water, it loses its good mechanical properties and swells, which forms a restriction in the range of potential applications. In the original paper by Yu et al., 2021, BMBC is proposed for applications in furniture and protective garments due to its recyclability. The capacity to be recycled is valuable, but it is also essential to tune the material’s water resistance properties according to the desired applications. In this report, different strategies for ameliorating water resistance properties of BMBC are discussed, and two such strategies, chitosan and potassium stearate coatings, are quantitatively evaluated.
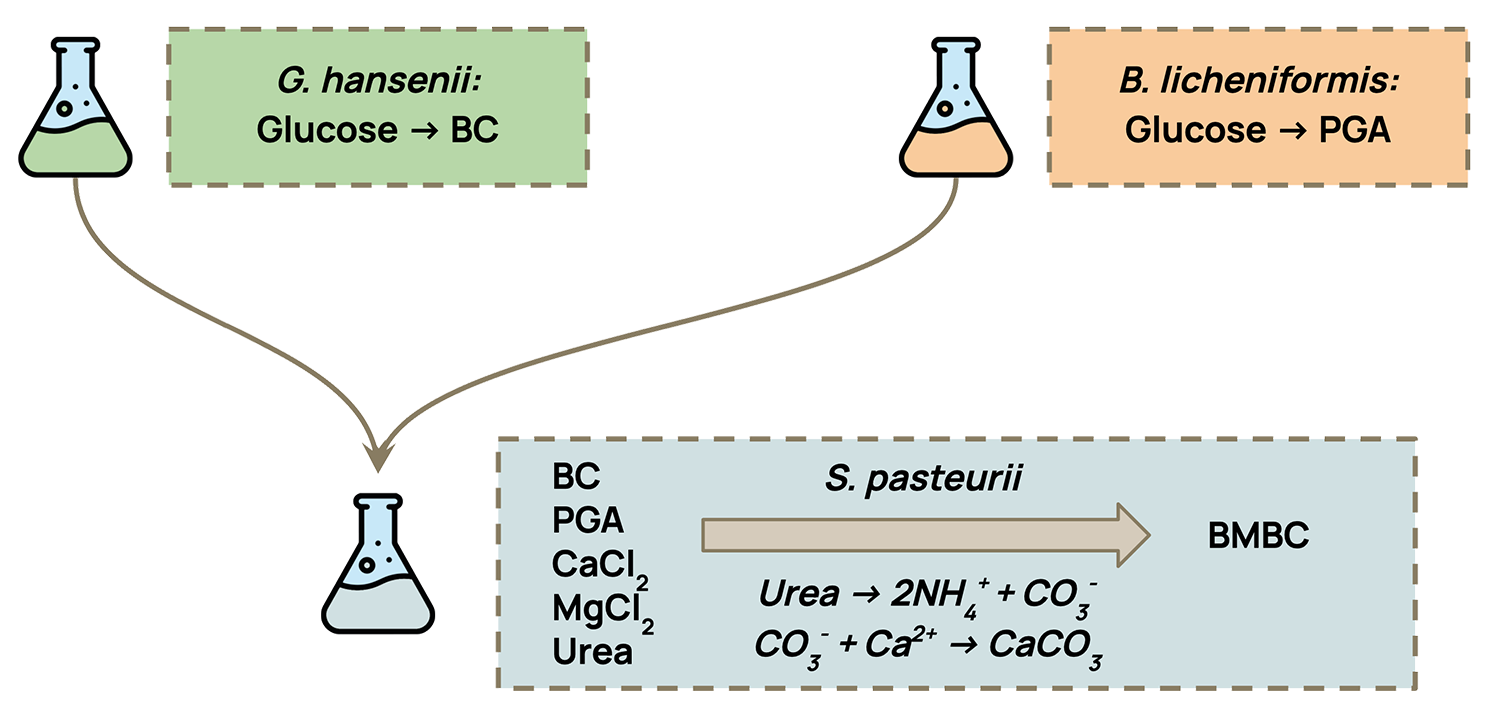
Figure 1. Schematic representation of the BMBC production process.
Figure 2a. Schematic representation of BMBC molecular structure.
Figure 2b. TEM micrograph of the BMBC surface under 5000x magnification.
Cellulose fibres, which make up 40% of the material, are wrapped along and around the spherical mineral crystals, which make up the other 60%.
Understanding hydrophobicity
The example of lotus (Nelumbo nucifera) leaves can demonstrate the two main strategies for ensuring surface hydrophobicity:
- Epicuticular waxes cover the leaves of this plant; these waxes are hydrophobic molecules and lower the surface energy of the leaf. Lowering the surface energy results in water molecules being more attracted to each other than to the molecules of the surface. Thus, water molecules stick together instead of wetting the material.
- Micropapillae cover the leaf’s surface, which leads to the formation of a very rough structure on micro-and nanoscales. Such microstructures lead to the inability of the water drop to come in contact with the surface of the material without overcoming a critical pressure, resulting in the preferential adherence of the water molecules to themselves.
Therefore, the two main strategies that can be employed are successfully modifying the surface of BMBC to have lower surface energy or inducing the formation of such microstructures.
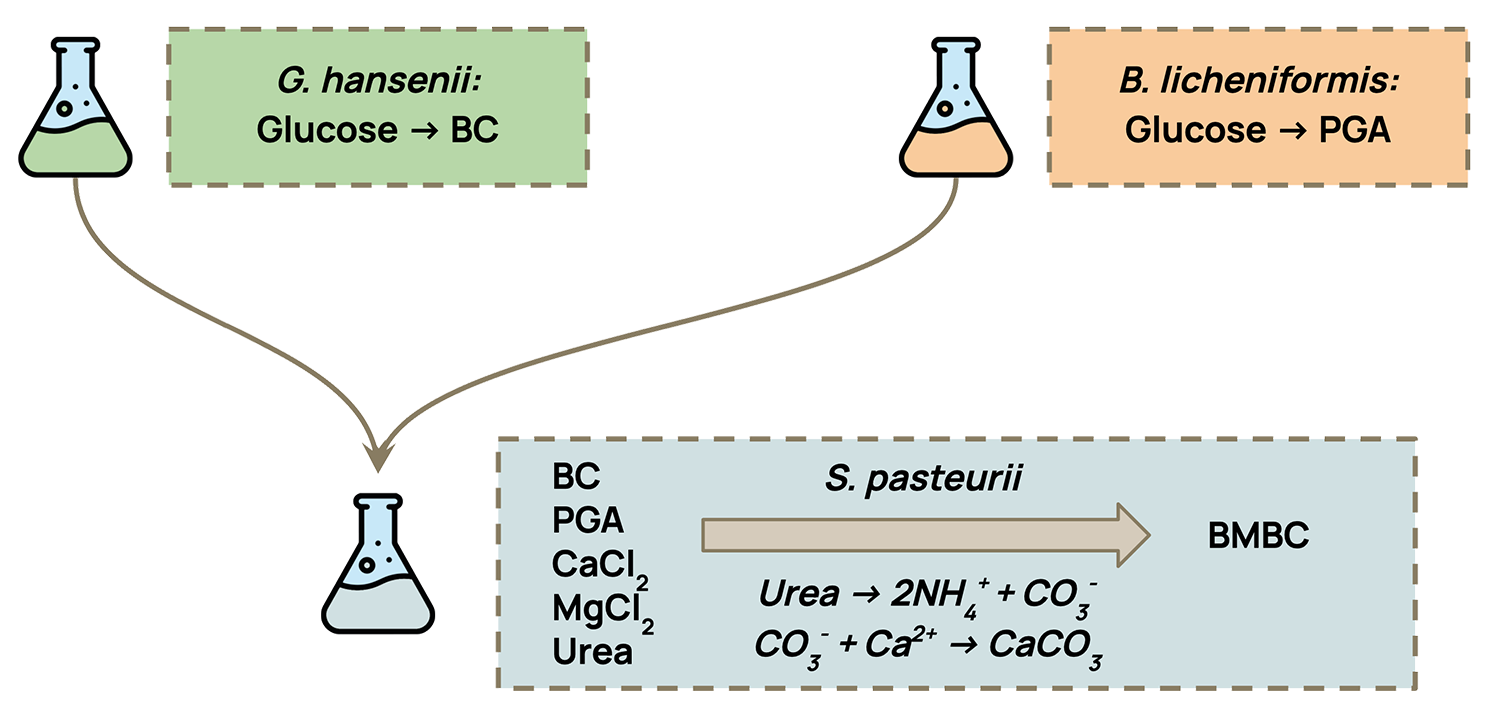
Figure 1. Schematic representation of the BMBC production process.
However, in a real life setting the measure of hydrophobicity does not necessarily equate to the ability to resist water. If an object is intended to be subject to touch or wear, we have to consider its wettability. If the material’s surface succeeds in preventing the water droplets from seeping through into the bulk of the material, we can speak of excellent absorption resistance. On top of that, the uniformity of the surface must be considered, as cracks and anchoring points can lead to deviations from an expected behaviour of (coated) the material surface.
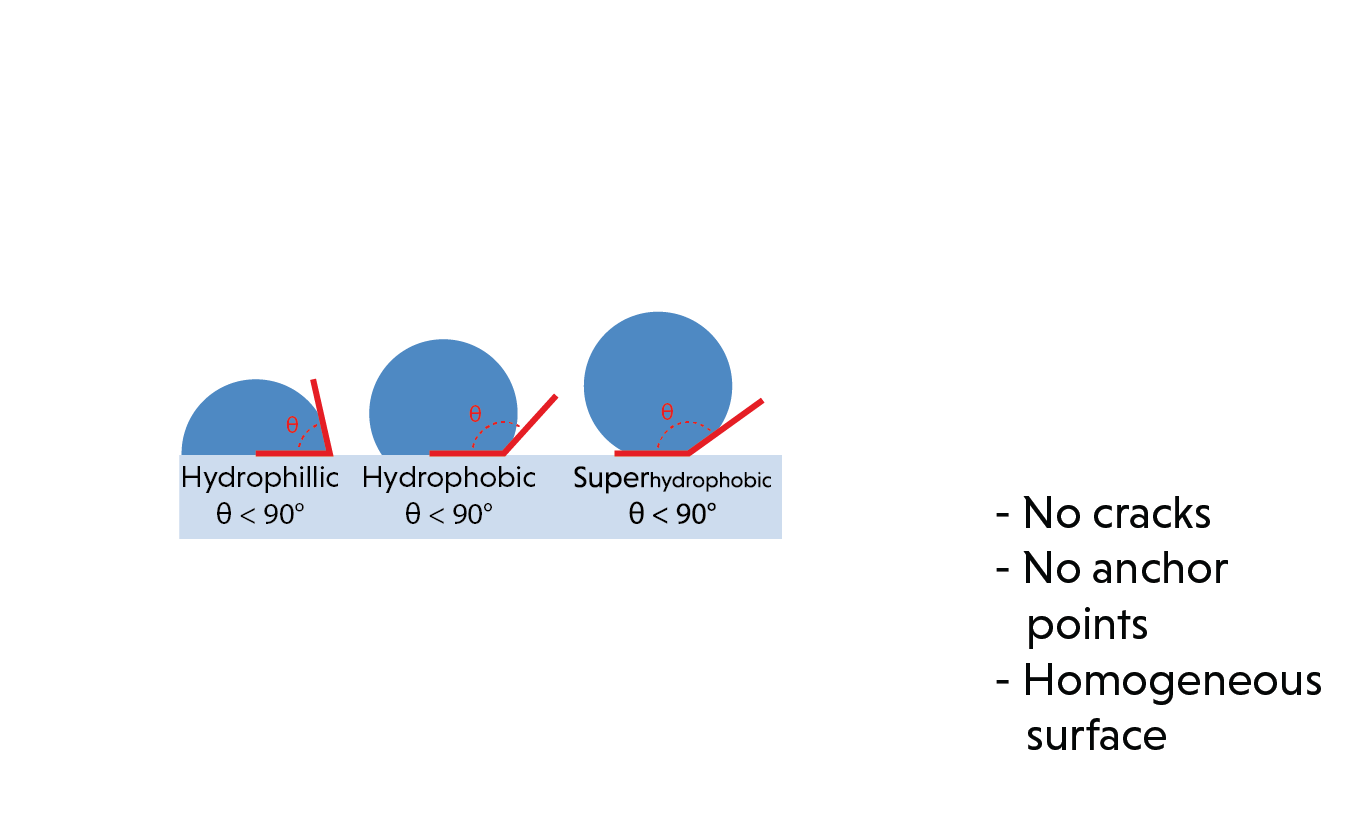
Experiments
Examples of compounds used for improving water- and oil resistance of paper and other cellulosic films include starch, beeswax, polydopamine, soybean oil and ZnO, zeolite, betulin, and many other biological or bio-derived molecules. However, many of these approaches utilise elaborate coating techniques, such as requiring special equipment.
The choice in favour of dip-coating and composite formulation was made to maintain the simplicity of BMBC formulation. Dip-coating can be conducted after drying the material to a sample of any size, which facilitates scalability. In addition, the biomineralisation process allows for incorporating other molecules into the BMBC composite, which can be used to tune the mechanical and physical properties of the material. Finally, three compounds that fit the selection criteria on application simplicity and biological nature were chosen to be tested on their capacity to enhance the hydrophobicity of BMBC: potassium stearate, chitosan, and zein.
Potassium stearate
The approach towards coating BMBC with stearate was based on Hu et al., 2009, and it drew particular interest due to the proposed ion exchange mechanism that stearate anions experienced upon contact with CaCO3. This mechanism was expected to highlight the benefits of the mineral composition of BMBC by utilising the present mineral crystals for surface grafting, resulting in increased hydrophobicity (also see Figure 1.2).
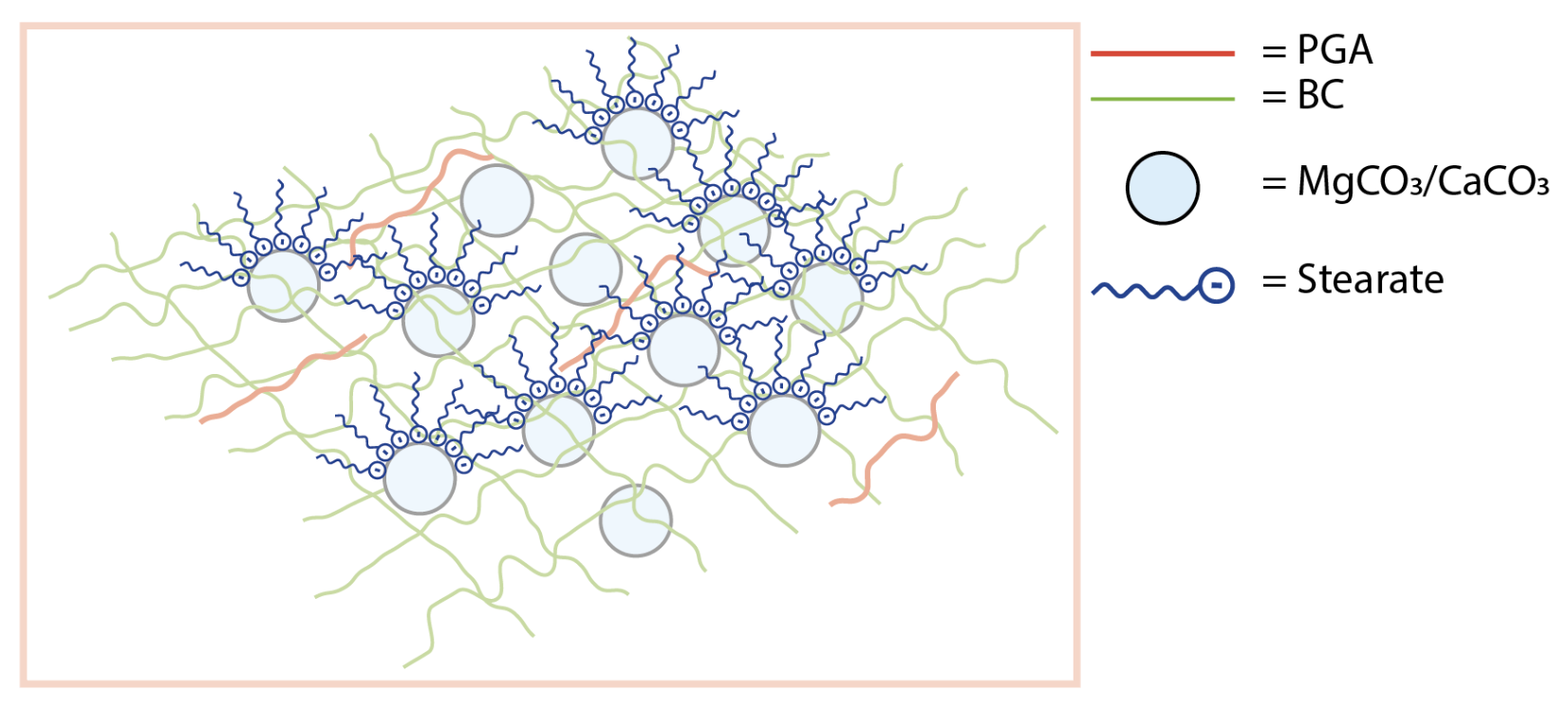
Figure 3. Schematic representation of the hypothetical mechanism of stearate anions’ adhesion to the surface of calcium carbonate crystals. This mechanism is supposedly responsible for surface grafting of BMBC, which manifests into high surface hydrophobicity of the coated material.
Chitosan
The second molecule that was selected for quantitative analysis is chitosan. Chitosan is a linear hydrophobic polysaccharide that is produced by exposing chitin to an alkaline environment (see Figure 1.3 for molecular structure). Chitin is the second most abundant polysaccharide in nature after cellulose, which makes chitosan an attractive candidate as a renewable and readily available substrate for hydrophobic coating. It also possesses antibacterial properties, consolidating the interest in integrating chitosan into the formulated BMBC material.
After dip-coating BMBC in chitosan, the sample is dried, and, as the chitosan coating loses the liquid, a chitosan film is expected to form on the sample’s surface. This film is expected to enhance the hydrophobicity of the surface using chitosan’s natural hydrophobicity and by increasing the roughness of the surface.
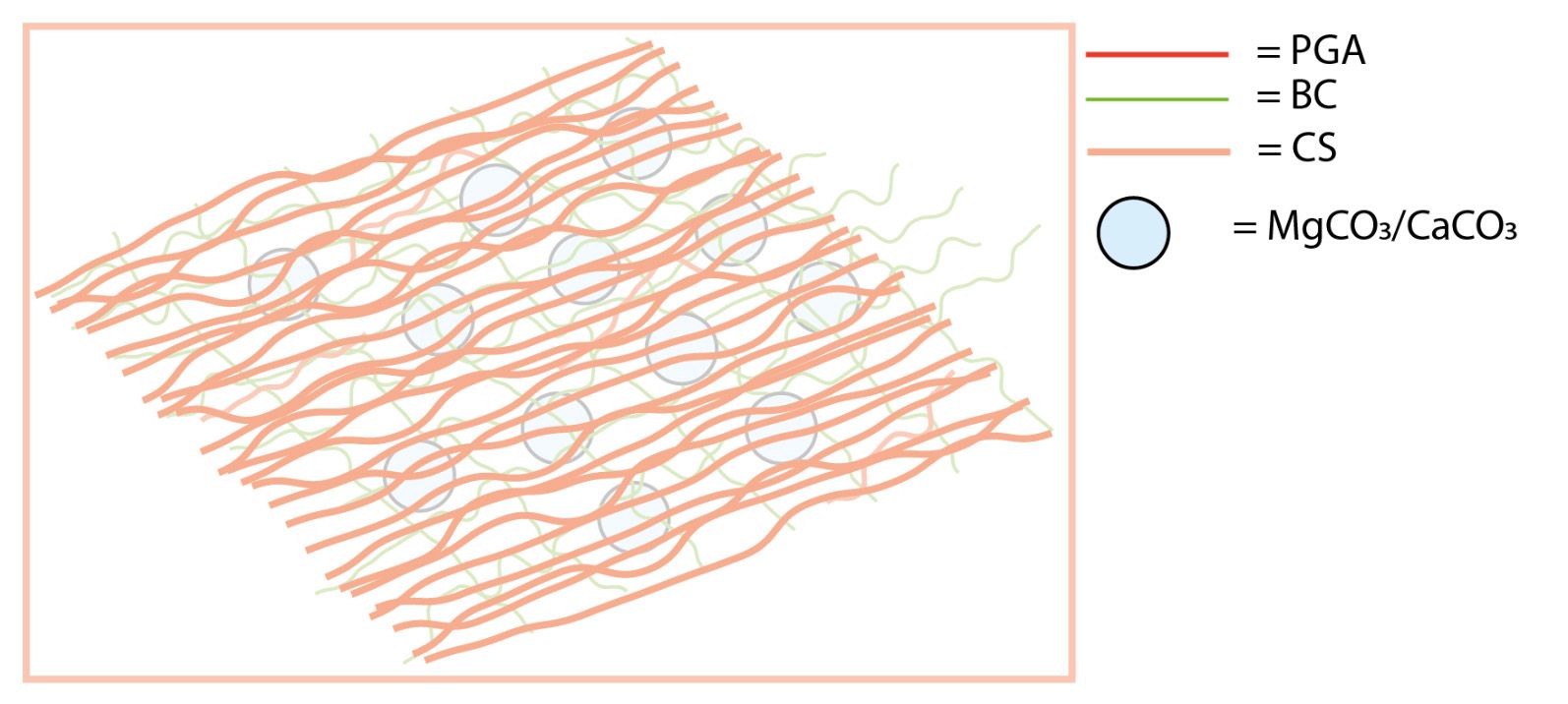
Figure 5. Schematic representation of the material’s microstructure upon formation of the chitosan film on the surface.
Zein
The third compound that was identified as a suitable substrate for enhancing the hydrophobic properties of BMBC was zein. This hydrophobic maize protein can be found in maize endosperm cells. It contains more than 50% of hydrophobic amino acids and has been employed in formulating nanomaterials with outstanding hydrophobic properties. Zein can also form various microstructures on the surface of materials when evaporation from this surface or the bulk of the material is induced, thereby increasing the roughness of the surface on a microscale. These microstructures would, in turn, decrease the surface’s wettability and improve hydrophobicity. At higher concentrations zein is expected to form a film similar to chitosan, as described above.
Figure 6. Schematic representation of the material’s microstructure upon formation of the a) zein microstructures and b) zein film on the surface of BMBC.
Coating robustness
In order to test the robustness of the applied hydrophobic coating, the surface’s hydrophobicity was tested after controlled abrasion of the sample. Abrasion was performed based on Veigel et al., 2017 and included applying a force of 2N onto a coated sample of 1.5×1.5cm and dragging for 30 cm along a sandpaper surface with a grain of 88 μm with a velocity of 10cm/s. Controlled abrasion was done using a 3D printer as a basis for the set-up that is depicted in the figure below. For results of this measurement, refer to the section 3.4.1 of the Full Thesis PDF.
Results
In this section, three graphs displaying the cross-comparison of the coatings’ performances is described briefly. For a more elaborate discussion, including potential explanations and implications, please refer to the Full Thesis PDF.
Water Resistance Level #1. Hydrophobicity
As discussed prior, the basic level of considering material’s ability to resist water is expressed as the angle that a drop of water stably forms on the surface of the material upon contact: Water Contact Angle (WCA). Figure 7 shows an example of a WCA measurement. Typically, starting at a WCA > 90°, a surface is considered to be hydrophobic.
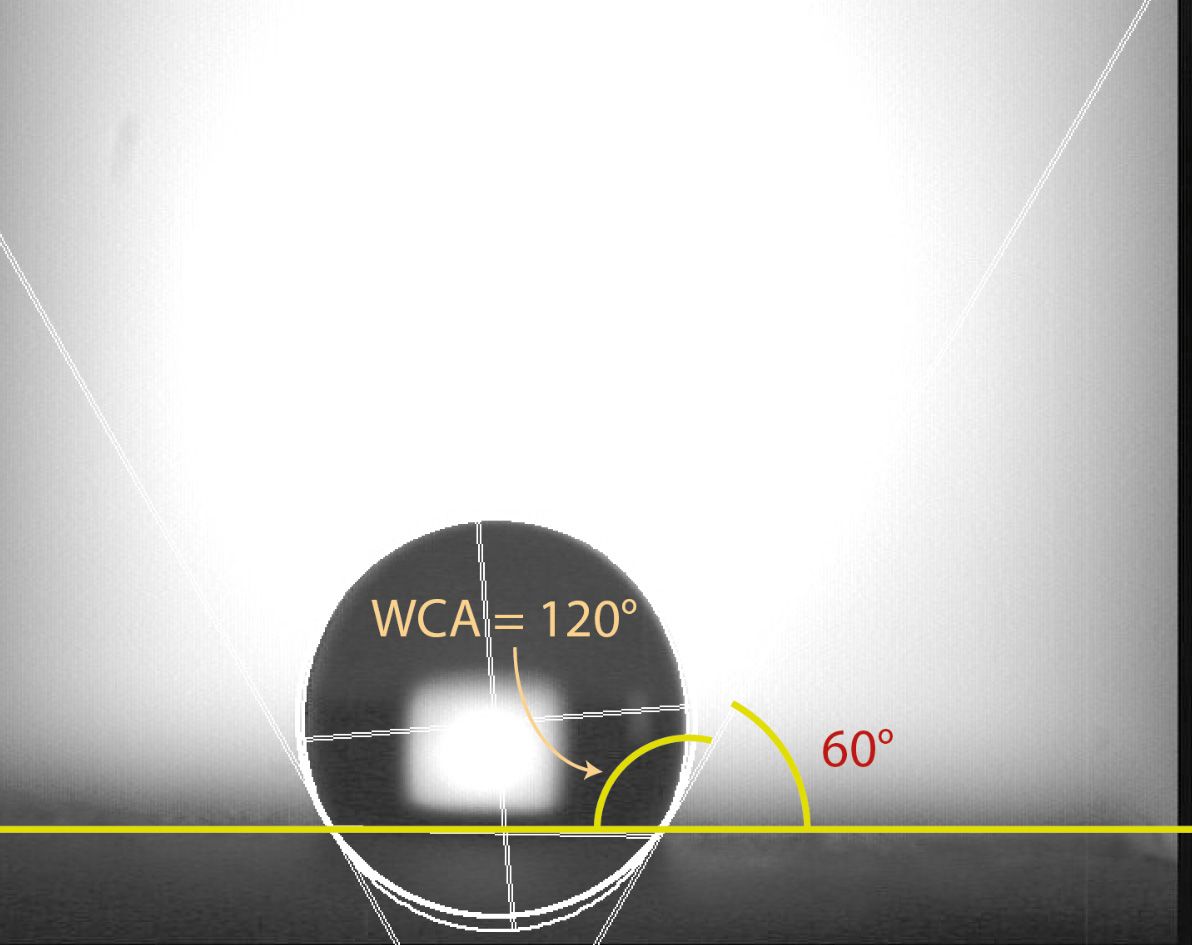
Figure 7. A schematic representation of Water Contact Angle (WCA) being measured using a macro lens on a sample of stearate-coated BMBC.
The results of WCA measurements for the three coatings at various concentrations are displayed in Figure 8. A few main takeaways are:
- Even though all coatings result in a significant increase in WCA when compared to the control samples, only stearate consistently results in the statistically relevant increase of the WCA to >90°.
- Longer immersion of BMBC into stearate results in better hydrophobicity.
- Chitosan-coated samples should be dried horizontally to achieve most effective hydrophobicity.
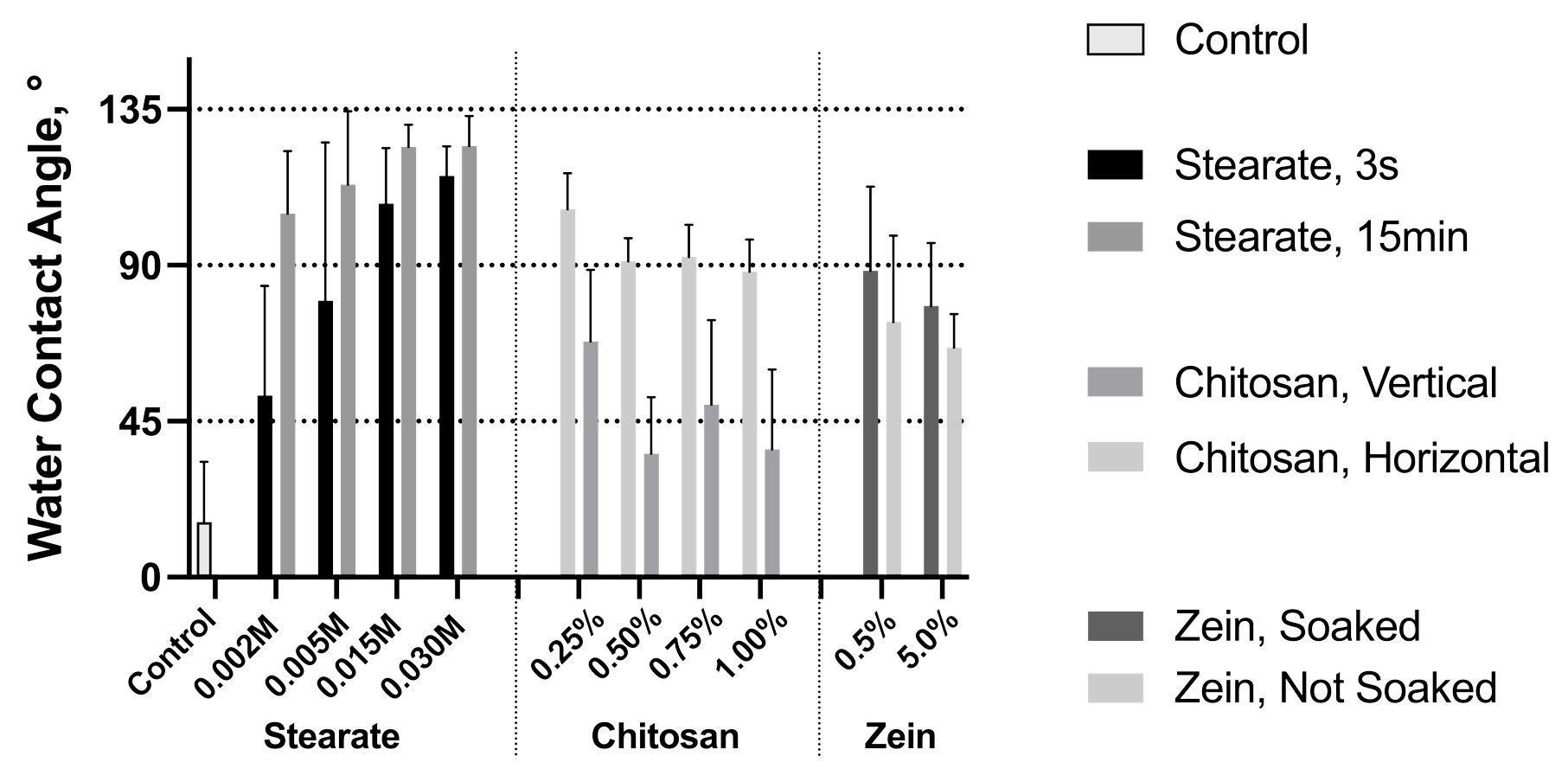
Figure 8. Water Contact Angle measurements for BMBC samples coated with stearate, chitosan and zein at varying concentrations and different methods of application.
Water Resistance Level #2. Water Drop Absorption
Another metric used to quantify the coating’s capacity to improve the wettability of the BMBC sample is Water Drop Absorption. During the experiments, it became evident that most coatings were not homogeneous enough to ensure robust sample encapsulation. Therefore, when a water drop is placed on the surface of the sample, it starts slowly seeping through. The time it takes for a drop of water to disappear from the material’s surface by either seeping through or evaporating was recorded. The theoretical value for evaporation of a water drop of 6μL was calculated. This value amounted to 69.4min, which roughly corresponds to the maximum value obtained for stearate: 72.5min. This correspondence points to the fact that the water drop did not seep through the stearate coating but instead evaporated.
The results are displayed in Figure 9. For this test, all coatings except for 0.25% chitosan and 5.0% zein yielded significantly better performance (p < 0.05) than the control.
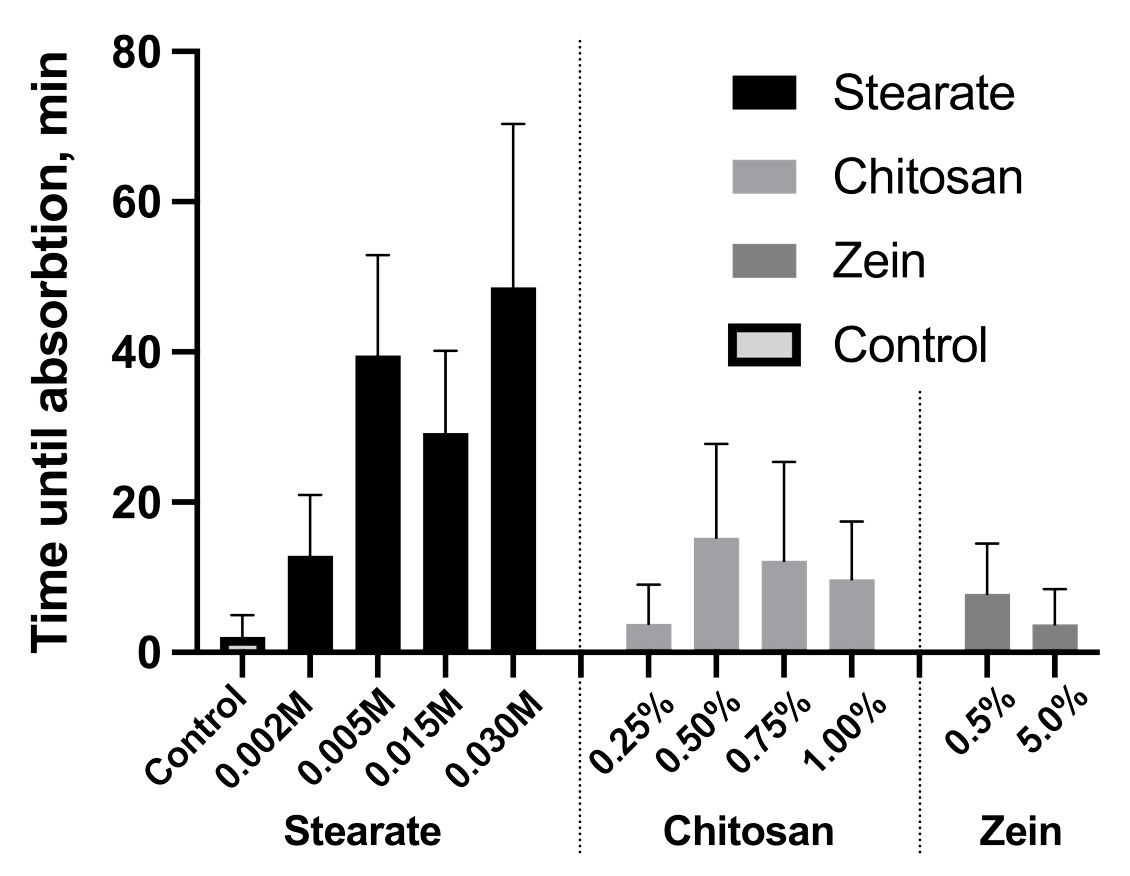
Figure 9. Drop Absorption measurements for BMBC samples coated with stearate, chitosan and zein at varying concentrations.
Water Resistance Level #3. Water Absorption Capacity
Finally, to evaluate the water-resistant properties of the coated samples,WAC measurements were performed over one hour and after 24h. WAC represents the ability of the coating to prevent wetting and water absorption when the sample is fully immersed in water. The results of these measurements are shown in Figure 10. The plot shows a more significant distribution of the WAC in the first thirty minutes, after which it converges towards the negative control measurements.
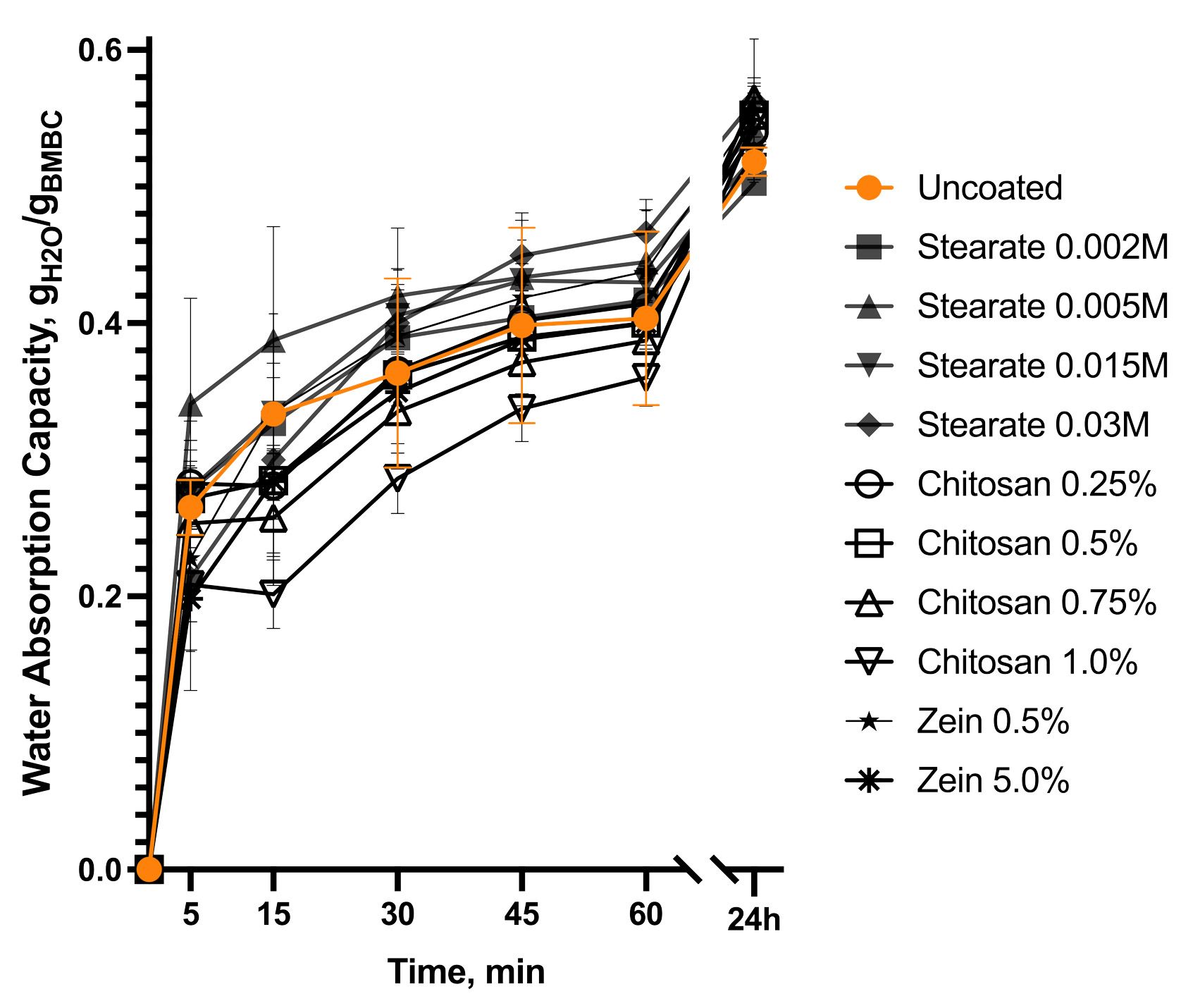
Figure 10. Water Absorption Capacity measurements for BMBC samples coated with stearate, chitosan and zein at varying concentrations. In the first hour the mass measurements are taken at 5, 15, 30, 45, 60 minutes, then after 24h.
Future prospects
Firstly, the current protocol for formulating samples of BMBC is not optimised for obtaining flat samples, which leads to the formation of highly porous and uneven surfaces when dried. Sometimes the obtained samples are so uneven that even thorough sandpapering does not provide sufficient flatness. Furthermore, when the samples are not flat before applying the coating, this could result in film cracking, as with chitosan or zein, or in the non-homogeneous distribution of surface-grafted stearate molecules on the surface. These features result in disturbed or unevenly distributed water-resistant properties of the coated samples, which results in inconsistent WCA measurements and poor performance in preventing water absorption since irregularities in the coating enable water to seep through the coating. This shortcoming of the current BMBC sample formulation protocol should be addressed if a more consistent methodology for any coating for BMBC is to be devised.
Secondly, the ion exchange mechanism should be elucidated to claim whether stearate has the highest affinity to MgCO3 and CaCO3 in BMBC. Then, perhaps, a different salt of stearate, e.g., sodium, will be more efficient in facilitating the ion exchange with the mineral crystals in BMBC, which would improve the water-resistant enhancing properties of the applied stearate coating. In general, this capacity of employing an ion-exchange mechanism for surface grafting of BMBC is an attractive topic for further investigation.
Besides stearate coating, chitosan and zein coatings also experience suboptimal performance, which can be attributed to the mode of application of the chosen coating, as well as the structure of BMBC’s surface. As seen in the TEM micrographs, the uneven nature of the BMBC surface causes disturbances in the formulated coatings. These disturbances, in turn, lead to poor performance in protecting the material against water absorption, which is detrimental to the water-resistant performance of the coated samples.
Another briefly discussed technique to enhance the water-resistant capacity of the
BMBC coating is the formulation of a chitosan composite. Even though a statistically significant increase in WCA was not achieved (p = 0.09), the occasional occurrence of WCA > 90° points to the potential of optimising this method to tweak the properties of BMBC.
To conclude, BMBC is a promising bio-based material with attractive mechanical properties but less advantageous physical properties. Further research into tweaking physical properties is required to achieve the appropriate tunability for desired applications.